A ketone and an enol differ from each other only in the location of a double bond and in the location of hydrogen. Such isomers are called tautomers. Tautomers are isomers differing only in the positions of hydrogen atoms and electrons; the carbon skeletons are the same. The conversion of one tautomer into an other tautomer is called tautomerization. If an aldehyde or ketone possesses at least one hydrogen atom on the carbon atom adjacent to the carbonyl group, called the alpha (α) carbon, this hydrogen can migrate to the oxygen atom. As a result, a carbonyl compound with alpha hydrogen can exist in two isomeric forms, called tautomers. In the keto form the hydrogen is attached to the alpha carbon, while in the enol form it is attached to the carbonyl oxygen with the migration of the double bond. Keto–enol interconversion is also called keto–enol tautomerization or enolization. The tautomers of acetaldehyde are shown here.
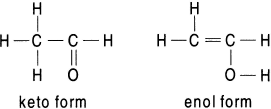
Acetone exists in equilibrium almost wholly in its keto form, as the enol form is not stabilized through either resonance or the intramolecular hydrogen bonding.
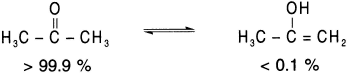
The enol form is given that name because it is a combination of ene for the double bond and ol for the −OH (hydroxyl) group. The two isomers exist in equilibrium. In any such case, both tautomers are present, but in simple cases the keto form is much more stable than the enol form. For example, in acetaldehyde about 6 molecules out of every 10 million molecules are in the enol form at any given time. Nevertheless, the equilibrium always exists, and every molecule of acetaldehyde (as well as any other aldehyde or ketone with an alpha hydrogen) is converted to the enol form (and back again) several times per second. This is an important characteristic because a number of reactions of carbonyl compounds take place only through the enol forms. The fraction of enol tautomer is considerably greater for a β-diketone because its enol tautomer is stabilized by internal hydrogen bonding and by conjugation of carbon–carbon double bond with the second carbonyl group. For example, in the case of 2,4-pentanedione, 80 of every 100 molecules are in the enol form.

Ethyl acetoacetate (CH3COCH2COOC2H5) gives the reactions of a carbonyl (C=O) group, such as the formation of cyanohydrin with HCN, bisulphate addition compound with sodium bisulphate and phenyl hydrazone derivative with phenyl hydrazine. In addition to these, it behaves as an unsaturated alcohol (enol) as it evolves hydrogen on treatment with sodium, shows reddish–violet colouration with ferric chloride and decolourizes bromine water and adds diazomethane. These chemical properties of ethyl acetoacetate suggest that it exists in two forms: a saturated ketone A (keto form) and an unsaturated alcohol B (enol form), which is an example of prototropy that can be shown as:
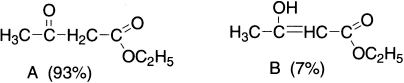
The hybridization of carbon flanked by carbonyl and ester groups changes from sp3 in A to sp2 in B. These two forms exist together so that properties of both keto and enol are observed in the reactions of ethyl acetoacetate. Structures A and B are isomeric and differ in the relative position of hydrogen atoms and the position of the double bond. Since a 1,3–proton shift takes place in the above example from carbon atom to oxygen atom, this form of tautomerism is known as prototropy. The enol form is favoured in relatively non-polar solvents such as alkanes and carbon disulphide and also by dilution. In hydroxylic solvents such as water, methanol, ethanol and acetic acid etc., the keto form is favoured due to the formation of hydrogen bonds between the oxygen atom of the carbonyl group and the hydrogen atom of the solvent molecule (ZH).
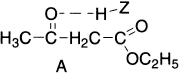
On dilution, the intermolecular hydrogen bonds (between two different types of molecules like A and ZH are broken and, therefore, the percentage of enol form in the mixture increases. Two factors usually stabilize the enol form.
- Intramolecular hydrogen bonding: Hydrogen bond formation within the same molecule as shown here in the enol form of 1,3–diketones.
- Resonance: The enol form (phenol) of either 2,4–cyclohexadienone or 2,5–cyclohexadienone (keto forms) is stabilized by resonance to a greater extent than a similar stabilization of the keto forms, which require charge separation. Phenol is unusual in that its enol tautomer is more stable than its keto tautomer, because the enol tautomer is aromatic but the keto tautomer is not.
Sometimes, because of resonance, the keto form is stabilized more as compared to the stabilization in the enol form due to intramolecular hydrogen bonding. This explains the larger percentage of the keto form as compared to the enol form of ethyl acetoacetate. The resonance effect is more pronounced in diethylmalonate where the keto form gets stabilized and hence only traces of enol are present.

In the liquid state, the percentage of enol forms in acetylacetone and benzoyl acetone is 80 and 89, respectively. In addition to intramolecular hydrogen bonding shown above for acetyl acetone, the enol form of benzoyl acetone gets further stabilization because of the conjugation of the carbon–carbon double bond with the phenyl group.
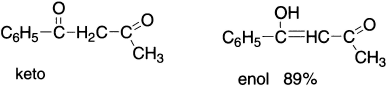
In a series of cis-fixed bicyclic diketones I, II and III the percentage enol varies from 100 to 80 and 1.4, respectively; in this series the hydrogen bond becomes obviously longer and weaker. For disubstituted 1,3-diketones (IV) the enol content increases rapidly as the size of the alkyl substituent is increased, when R is tert-butyl, the keto form is undetectable.

The keto-enol equilibrium has often been used as a measure of the resonance stabilization; for example, no enolization occurs in 3-cyclobutenones (V) and no ketone can be detected in phenol (VI). Fluoroglucinol (VII) chemically behaves more like a triketone than a triol; thus, it readily forms the trioxime (VIII) with hydroxylamine.
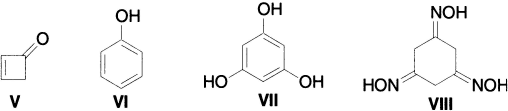
Sometimes, conformation plays a dominant role in deciding the extent of enolization in certain α-diketones. Biacetyl, for example, has very little enol content in contrast to 1,2-cyclopentanedione, which exists mostly in the enolic form. The carbonyl groups of the biacetyl are oriented in opposite directions so as to reduce dipole–dipole repulsion, whereas cyclopentane-l,2-dione (C) being a cyclic compound has no such choice and has to resort to enolization in order to avoid this repulsion.
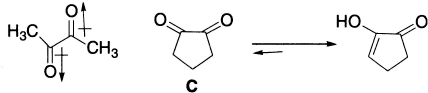
These are some examples of keto–enol tautomerism. Besides these, some other systems also exhibit tautomerism.
Mechanism of Keto–Enol Interconversion
Keto–enol interconversion may happen in basic as well as acidic solution. The steps are reversed in the base- and acid-catalyzed reactions. In the base-catalyzed reaction, the first step is the removal of an α-proton and the second step is the protonation of oxygen. In the acid-catalyzed reaction, the first step is the protonation of oxygen. In the acid-catalyzed reaction, the first step is the protonation of oxygen and the second step is the removal of a α-proton.
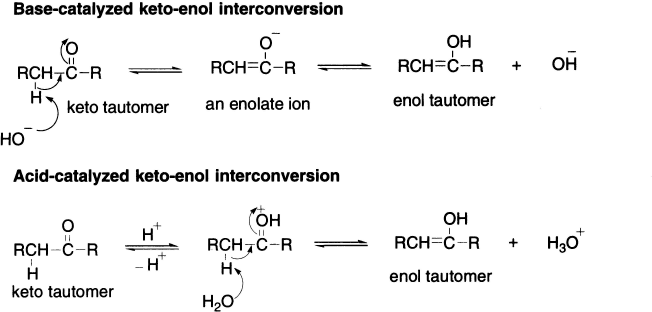
Differences between Tautomerism and Resonance
- Tautomerism usually involves making and breaking of σ as well as π bond. While in resonance, only the electrons in π bonds or lone-pairs on heteroatoms shift; the σ framework is not disturbed. This difference results in a shift of an atom from one position to another in tautomerism. In resonance there is no shift of any atom.
- Tautomerism may involve a change in hybridization of atoms resulting in a change in shape of the molecule. In resonance there is no change in hybridization or the geometry.
- The two tautomeric forms exist together though the equilibrium may shift to either side with a change in condition. The tautomers have a physical reality whereas the resonance structures are imaginary.
Leave a Reply